Li-S Battery Electrode with TiO2-TiB2 Composite Additive for Polysulfide Shuttling Mitigation
Nov 30, 2019
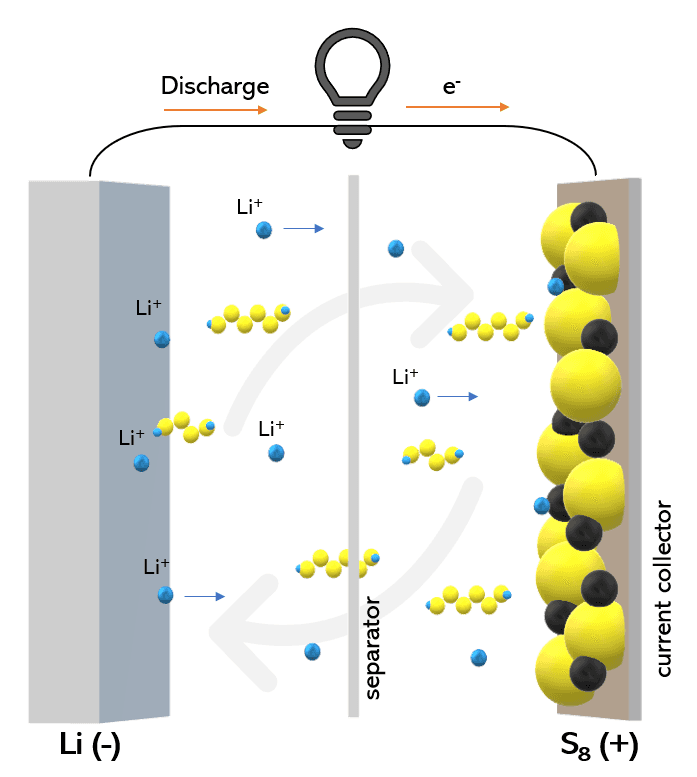
This is from my unpublished paper on m y work done in 2019 and 2020 where I explored nanomaterials to enhance the performance of Lithium-Sulfur batteries.
Abstract
Lithium-Sulfur batteries are one of the most studied next generation energy storage devices due to their large energy density and economic advantages due to the low cost of sulfur, but suffer from poor cycle life due to the dissolution and shuttling of polysulfide species during the charge-discharge process. Polysulfide shuttling mitigation has been explored in a variety of ways over the past few years. Here, we report the synthesis of a novel TiO2-TiB2 composite from bulk TiB2 powder for polysulfide shuttling mitigation. The combination of the polar effects of TiO2 and conductivity enhancement by TiB2 enables excellent polysulfide anchoring and aids in efficient conversion of polysulfides to Li2S, providing a stable cycle life even at 1C, with an energy density of ~750mAhg-1 after 250 cycles.
Introduction
With the rapid advancement of renewable energy technologies, long term and stable energy storage is becoming ever more critical. Rechargeable lithium-sulfur batteries hold promise in applications where large energy density is paramount. They have a specific capacity of 1675 mAh/g and an energy density of 2600 Wh/kg. This is much higher compared to lithium-ion batteries which have a maximum energy density of ~400 Wh/kg. Lithium-sulfur batteries, however, suffer due to low conductivity of sulfur (5 x 10^-30 S/cm), volume expansion of the cathode (~80%) and the shuttling of intermediary soluble polysulfides formed during the charge-discharge process, which lead to parasitic reactions, poor sulfur utilization and poor cycle life, preventing them from widespread usage.
Many strategies have been employed to enhance the performance of Li-S batteries, among which the performance enhancement of the cathode has been the most widely studied. While physical confinement of polysulfides using high surface area porous structures such as mesoporous carbon and hollow carbon spheres was one of the early strategies to increase performance, the polysulfides would unavoidably leak out and contribute to low cycling performance. To counter this, the polysulfides must be prevented from dissolving in the electrolyte, by anchoring them to the cathode itself. In this regard, nitrogen doped carbon materials enhance the affinity of the polysulfides due to the electron donating property of nitrogen which results in an affinity towards polysulfides. But due to the low percentage of nitrogen doping, there is an upper limit to the number of anchoring sites that attract polysulfides.
To achieve this, polar inorganic materials have been studied to anchor these polar polysulfides on to the cathode itself, preventing the shuttling from occurring and increasing the efficiency of the battery. Transition metal oxides like titanium oxides (TiO, TiO2 and Ti4O7), manganese dioxide (MnO2), iron oxide (Fe3O4), niobium pentoxide (Nb2O5) and silicon dioxide (SiO2), borides like magnesium boride (MgB2) and titanium boride (TiB2), carbides like titanium carbide (TiC) and niobium carbide (NbC) and sulfides such as molybdenum disulfide (MoS2) and tungsten disulfide (WS2) have been widely studied for their polysulfide shuttling mitigation capabilities. Most polar compounds, however, have low electrical conductivity, resulting in low efficiency of electron transport and poor usage of sulfur. The synthesis of hybrid nanostructures exhibiting both good conductivity as well as polar behavior is therefore extremely useful for efficient functioning of Li-S batteries.
Titanium diboride is a widely available material possessing high electronic conductivity (~105 S/cm) which is useful for accelerating polysulfide conversion reactions and has a good polarity for polysulfide shuttling mitigation and homogeneous precipitation of Li2S. TiO2 on the other hand has a much higher chemical polarity than TiB2 and has been more widely studied but suffers from poor conductivity. Coupling these properties would result in a superior hybrid material capable of efficient shuttling mitigation.
Here, we report the synthesis of a novel TiB2-TiO2 hybrid nanostructure that is used as the polar additive in sulfur cathodes. The synergistic combination of nano TiO2 and conductive TiB2 exhibit the following advantages: 1) conductive and polar TiB2 provides a framework for efficient polysulfide conversion and uniform Li2S precipitation 2) the polar nano TiO2 enables highly efficient polysulfide anchoring 3) the resulting chemical and physical entrapment (enhanced by addition of graphene) enables highly efficient polysulfide anchoring and conversion, 4) the simple synthesis process involves heating of TiB2 powder in a highly environmentally friendly deep eutectic solvent in an autoclave which also results in ease of scalability. The electrochemical performance was highly superior with a capacity of ~700 mAh/g after 350 cycles at 1C and excellent rate capability at high currents up to 5C. Furthermore, we have extrusion printed these electrodes to achieve high mass loading of about ~5mg/cm^2 and have obtained excellent electrochemical performance. To the best of our knowledge, this is a completely new synthesis route for the TiB2-TiO2 nanostructure and there is no report on using this hybrid material as a polar host in Li-Sulfur batteries.
Electrochemical Performance
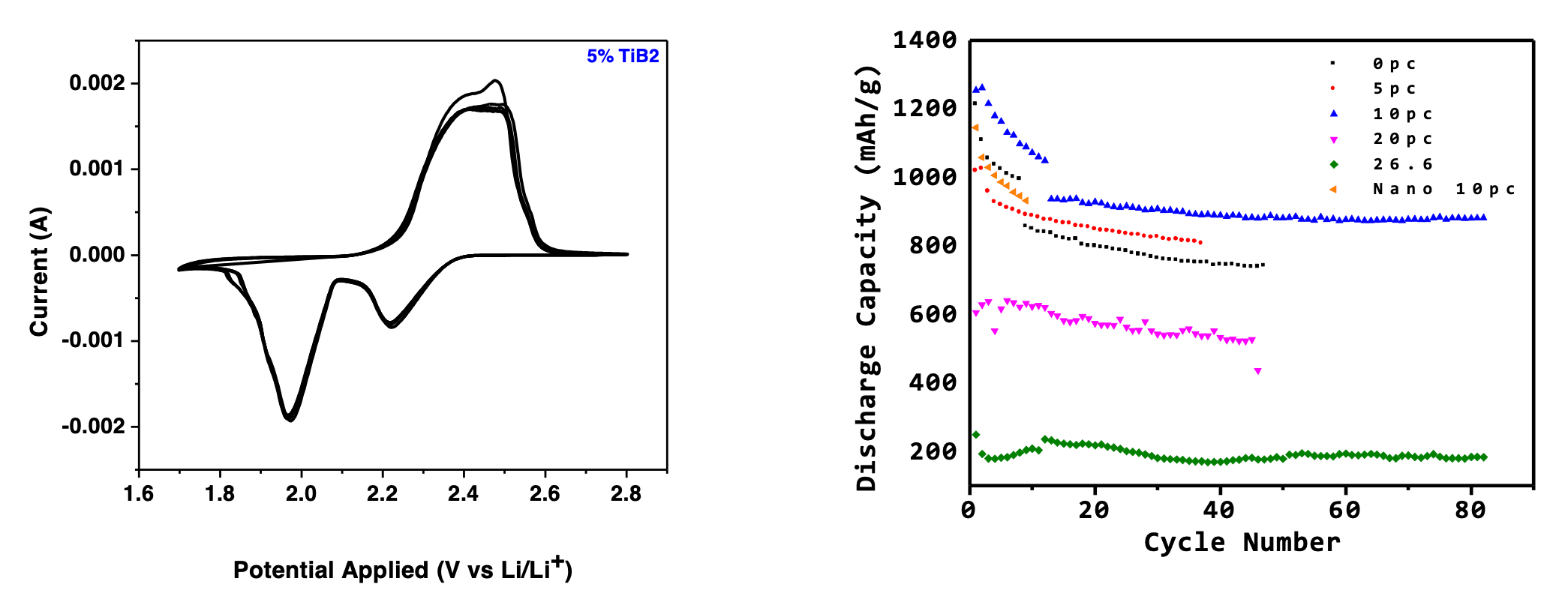
Electrochemical testing was done in a coin cell setup with lithium metal as the anode. The typical CV scans are shown in Figure 4a. A scan rate of 1 mV/s was employed. The cathodic scan showed two reduction peaks at 2.27 V and 1.93 V which is the result of elemental S8 decomposing into long chain polysulfides of the Li2Sn nature where 1<n<8. The final product formed is Li2S at the low potential. Two oxidation peaks at 2.43V and 2.53V are attributed to the conversion of short chain polysulfides to sulfur.
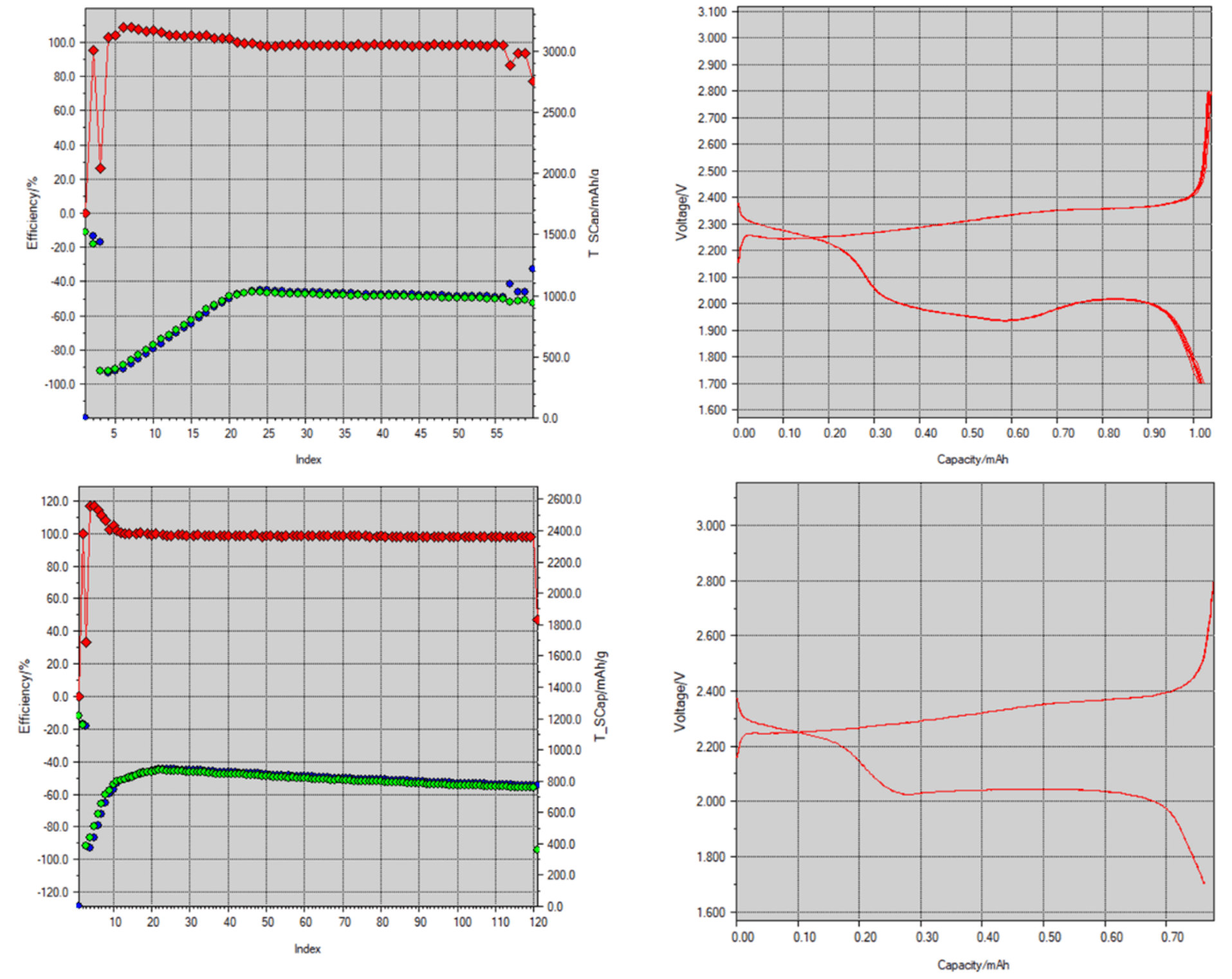
Galvanostatic charge-discharge profiles were obtained by running the assembled cells at varying current rates between 1.7-2.8V. To compare the electrochemical performances of the different materials used in this study, we first assembled cells with and without bulk TiB2 powder. As recently demonstrated by Li et al, the cells with TiB2 additive showed enhanced electrochemical performance with a capacity of about 800 mAh/g compared to cells without any additive. One reason is attributed to the B- honeycomb lattice in the crystal structure being isoelectronic to graphite, which provides rapid conductive paths for electrons and ions. Its hardness also plays an important role in suppressing the effects of sulfur volume expansion. We tested cells with varying quantities of TiB2 additive (0 to 30% with respect to sulfur) as detailed in the supplementary section and we found the optimal additive amount to be at about 10%. However, the electrochemical performance is likely to increase by using a more polar molecule in conjunction with TiB2 as discussed earlier. The electrochemical performance with this hybrid material is detailed in Figure. The cell was first cycled at 0.1C which showed an initial discharge capacity of ~1200 mAh/g which is about 71% of its theoretical capacity of 1675 mAh/g. After 250 cycles at 1C, it still showed a capacity of ~700mAh/g.
Experimental Section
Preparation of TiB2-TiO2 heterostructure. The deep eutectic solvent was prepared by dissolving 26.5g of choline chloride in 23.5g of ethylene glycol. After complete dissolution of the salt, 500mg of TiB2 powder was dispersed in the solution under constant stirring. This was then sealed in a 30mL autoclave chamber and heated at 180°C for 24 hours. The product was then washed with DI water and acetone and dried in a vacuum oven at 80°C overnight.
Preparation of TiB2-TiO2-Sulfur composite. Sulfur is loaded on to the hybrid material in a ratio of 7:3 by weight by hand milling followed by melt diffusion at 155°C for 12 hours.
Electrochemical measurements. The slurry for coating was made using the TiB2-TiO2-Sulfur composite, graphene and polyvinylidene difluoride (PVDF) in an 8:1:1 ratio by weight respectively. The PVDF was dissolved in a small quantity of N-methyl-2-pyrrolidone (NMP) before adding the other components. The slurry was coated on stainless steel spacers and dried at 80°C in vacuum overnight. The average sulfur loading of the cells were between 1-1.5mg/cm-2. CR2032 coin cells were used to fabricate the devices with lithium metal as the anode, Celgard 2400 separator and 1M LiTFSi in DOL/DME (1:1 v/v) with 2% LiNO3 was used as the electrolyte. Fabrication was done in an Argon-filled glove box with electrolyte loading of 10mL/mg sulfur. Electrochemical measurements were done on a LANHE battery system between 1.7 and 2.8V. Current rates were calculated using the specific capacity of sulfur. CV measurements were done on Autolab electrochemical workstation at 1mV s-1 while electrochemical impedance spectroscopy was done between 0.01Hz and 100 kHz. All experiments were conducted at room temperature.